In this issue of NanoNews-Now Editor Rocky Rawstern covers self-assembly via interviews with Christine Peterson (Foresight Institute), Chris Phoenix (Center for Responsible Nanotechnology - CRN), K. Eric Drexler (Foresight Institute), and Darren W. Johnson (University of Oregon).
Off the main topic: Dr. Pearl Chin (in the next in her monthly series) contributes an article titled What Is Happening to the Funding for Commercializing Nanotechnology?
Last, we reprint a pertinent article titled Many Options for Molecular Manufacturing from CRN
Join us as we present "Self-Assembly"
Find the nanotech patent information you are looking for faster, easier, and in greater detail than any other publication - GUARANTEED.
Tired of wasting countless hours searching for interesting new developments in nanotech that should be easy to find? If you are looking for up to date information on who is actively involved in filing nanotech intellectual property, then the NanoTech Transfer Report could be the most important time saving report you will ever read.
By reading the NanoTech Transfer Report you can:
* Keep up to date on important nanotech developments
* Discover interesting new nanotech patents
* Find lucrative partners before someone else does
* Save valuable research time
* and much more...
"The NanoTech Transfer Report provides me with the information I need to stay in touch with the leaders in the field. Great product!"
Douglas W. Jamison, Vice President Harris & Harris Group Inc. www.hhgp.com
|
A year's subscription includes 12 issues of our monthly report published on the last Friday of the month, and access to our patent database.
NanoTech-Transfer Patent Database
Full search Database - search by tech-sector, inventor, institution, application, patent number or date.
NanoTech-Transfer Report
Each monthly issue of the NanoTech Transfer Report delivers detailed information on the latest nanotech patents and patents pending from individuals, universities and research institutes from around the world.
Try it FREE for 90 days! There is NO RISK.
The NanoTech Transfer Report is only $39.95 per month - but wait! We know we have a great product, and we are willing to stand by it. Nanotechnology Now would like to offer you the chance to try it out for FREE. There is absolutely no risk to you for trying the NanoTech Transfer Report. Once you subscribe you will be given a login and password to review the report for free for the next 90 days. This gives you plenty of time to review the report in detail with no risk.
Click Here For Details
|
|
Table of contents:
Christine Peterson
Chris Phoenix
K. Eric Drexler
Darren W. Johnson
Pearl Chin
Quotes
Useful Links
Next Issue
Glossary
NanoStrategies
NanoTech-Transfer
NanoNews Digest
About Us
Contact
NanoNews-Now Editorial Calendar
|
Jan '05 |
Patents |
Tentative Schedule 2005 |
Feb '05 |
MNT |
Mar '05 |
Memory and Chip Tech |
Apr '05 |
Jobs |
May '05 |
Education |
Jun '05 |
Tools |
Jul '05 |
Investments |
Aug '05 |
Security |
Sep '05 |
Life Extension |
Oct '05 |
Military |
Nov '05 |
Materials |
Dec '05 |
Possible Futures |
|
Molecular Manufacturing Vs Molecular Self-Assembly
Christine Peterson, Founder and Vice President, Public Policy, Foresight Institute.
|
|
Questions by Tyler Emerson of the Singularity Institute
1. What is self-assembly?
One can imagine two basic ways to manipulate matter. One is the way we do it as human beings at the macro scale, which is direct manipulation; we pick things up with our hands, with our machines; we physically bring them together using 3-dimensional control.
The other way is self-assembly, which is the method that nature uses, down at the nanoscale. Taking place in a liquid medium, the parts that need to be brought together jumble about due to thermal activity, and come together in random configurations until they match up in terms of their shape and their electrical charges, and hold together. That's how nature builds down at the nanoscale.
The goal of course is for humans to be able to build new things at the nanoscale. So how can we do that? What we would ideally like is to combine these two methods so that we have the manipulation abilities that we have at the macro scale, down at the nanoscale. We have that using scanning probe microscopes now, but there's a huge drawback - the self-assembly method has millions or billions or trillions of actions occurring in parallel (it has massive parallelism). In comparison, scanning probe microscopes have little or no parallelism
So the goal is to combine these two methods down at the nanoscale.
2. How does self-assembly relate to molecular manufacturing?
The goal of molecular manufacturing is to build large products to atomic precision. This will require massive parallelism in terms of how molecules and then later larger sub-components are brought together to form a large object. Currently we are not able to get this kind of massive parallelism using the direct manipulation techniques we have available today at the nanoscale; primarily scanning probe microscopes.
Although work is being done to develop parallel SPM's, they're nowhere near the parallelism you get with self-assembly. So what we need to do to reach molecular manufacturing is to bootstrap off using self-assembly methods - those that nature has shown us work so well at building macro scale objects. We need to find development pathways that lead from techniques that primarily depend on self-assembly, to those which are mixed, and then finally those that have both massive parallelism and direct manipulation at the nanoscale.
When we get there we will have what is needed to do molecular manufacturing.
3. The nanotechnology bill recently passed by congress (link) included a study of molecular manufacturing, but at the last minute the wording of the study was changed from "molecular manufacturing" to "molecular self-assembly." Is this an issue?
We at Foresight were very pleased that molecular manufacturing studies were included the bill, but were disappointed when one of the studies was changed at the very last minute - the wording was changed from molecular manufacturing to molecular self-assembly.
The reason this is a problem is that molecular self-assembly is an extremely broad term that includes large swaths of work in other fields. So there was in fact no need to do a study of the feasibility of molecular self-assembly since it's already obviously feasible; it's done continually both by nature and artificially.
We were concerned when this wording change was made. However, our understanding is that the committee that is setting up the study was similarly confused by the new wording since it now makes no sense. They are taking a look at the legislative history to try to figure out what Congress could possibly have meant by this, and is finding pretty clearly what was meant, which is molecular manufacturing.
We have reason to hope that the study will go forward as was originally planned, and consider the feasibility of molecular manufacturing.
Christine Peterson writes, lectures, and briefs the media on coming powerful technologies, especially nanotechnology. She is Founder and Vice President, Public Policy, of Foresight Institute, the leading nanotech public interest group. Foresight educates the public, technical community, and policymakers on nanotechnology and its long-term effects.
She lectures on nanotechnology to a wide variety of audiences, focusing on making this complex field understandable, and on clarifying the difference between near-term commercial advances and the "Next Industrial Revolution" arriving in the next few decades.
Bio.
|
NN: What is the difference between molecular nanotechnology (MNT) and molecular self-assembly?
The most important difference is that self-assembly is not computer-controlled, so its output is limited in complexity.
In self-assembly, the complexity of the output is limited to the complexity of the physical inputs. Chemicals are mixed together and find their own way to join up into larger structures. The output cannot be any more complex than the component chemicals and the processes used to mix them. Some self-assembling chemicals such as synthesized DNA may be somewhat complex, but still can't build large-scale heterogeneous engineered structures.
In molecular nanotechnology (also called molecular manufacturing), the assembly is guided by computer-controlled nanoscale equipment. That means that the complexity of the output can be increased by feeding in new computer instructions, and a relatively simple manufacturing system can build products that have greater physical complexity.
Another difference is that self-assembly has to take place in some solvent so that the molecules can float around randomly. This increases drag, slowing the process. Molecular manufacturing should be able to do some if not all of its operations in gas or even vacuum, allowing the process to work faster.
NN: What forces were behind the replacement of a study of MNT with one for molecular self-assembly in the 21st Century Nanotechnology R&D Act? How does this change effect progress towards MNT in the U.S.? Why are you concerned that this change may have a negative impact?
As Howard Lovy documented (1), the NanoBusiness Alliance lobbied the bill's compromise committee for the change. Altering one word--molecular manufacturing vs. molecular self-assembly--changed the meaning drastically. Also, several proposed technical studies were cut entirely. The purpose was apparently to turn attention away from molecular manufacturing to the less powerful self-assembly.
Molecular manufacturing is quickly becoming accepted as a goal of nanotechnology--except by the U.S. government. There have not been enough studies to say exactly how hard it will be to develop, but it's safe to say that it is rapidly becoming easier. I'm concerned that if molecular manufacturing continues to be deliberately ignored, its policy implications will not be studied until the technology is actually developed, and that may be too late.
Direct computer control of molecular fabrication and assembly leads to a very powerful and scalable manufacturing technology. It seems likely to develop fast enough to create instability in geopolitics, economics, and society. We need to understand this in advance in order to have any hope of making wise policy. Unfortunately, the U.S. government (encouraged by certain nano-business interests) is not even looking--and worse, they're telling everyone else not to look.
(1) See Omission in the 21st Century Nanotechnology Research and Development Act
Chris Phoenix Director of Research, the Center for Responsible Nanotechnology. A non-profit organization, formed to advance the safe use of molecular nanotechnology, CRN was founded by Chris Phoenix and Mike Treder in December 2002. The vision of CRN is a world in which nanotechnology is widely used for productive and beneficial purposes, and where malicious uses are limited by effective administration of the technology.
CRN is a Nanotechnology Now "Best Of" Award winner, having won the "Best of the Best" and "Best Advocate" for the year 2003. See Best Of The Best 2003 for details.
Chris can be contacted here.
|
Last week we had some fun with the recent nanotech bill in the US, especially the plan for a one-time study to determine the feasibility of making things using molecular self-assembly, which makes about as much sense as conducting a one-time study into the feasibility of sharpening a stick with a sharp knife.
Paul Holister. Former Chief Architect of the Nanotechnology Opportunity Report, and editor of TNT weekly.
|
How self-assembly may play a role in developing Molecular Nanotechnology (MNT)
Dr. K. Eric Drexler
|
|
Molecular Nanotechnology (MNT): Thorough, inexpensive control of the structure of matter based on molecule-by-molecule control of products and byproducts; the products and processes of molecular manufacturing, including molecular machinery. (Foresight Institute)
|
Progress toward MNT means progress in building productive molecular machine systems -- advancing through a series of steps in which each generation of tools can build tools of the next generation. How can this process start?
It already has. Laboratory researchers today can design and make atomically precise nanoscale components from polymeric materials. Some of these materials are as strong and stiff as polycarbonate and epoxy resins. Like epoxy, these polymers can be used to bind together particles and fibers of other materials -- stronger, stiffer, more diverse materials -- to form composite structures. If the parts are atomically precise and designed properly, they can self-assemble in solution to form large, complex structures, including machines.
These polymeric materials can self assemble under laboratory conditions to form productive, digitally controlled molecular machine systems. This is not speculation, but fact. The polymers are proteins and nucleic acids. The machines are ribosomes. The digital control comes from the genetic system, via messenger RNA. Self-assembly of ribosomes in vitro from separate, purified molecular components was first demonstrated decades ago.
Molecular designers including Feynman prize winners Nadrian Seeman, Homme Hellinga, and David Baker have developed molecular CAD systems, and with them have designed and fabricated atomically precise components made of DNA and proteins. A natural goal for MNT development is to use the molecular machinery of ribosomes and DNA polymerases to make components designed to self assemble, forming next-generation productive molecular machine systems. These can be designed to make a wider range of materials, and thus a wider range of systems – including better tools of the next generation. This will continue the spiral of capabilities that leads from the chemistry and molecular biology of the past, through the biomolecular engineering of today, to advanced productive nanosystems of the future.
For the next stages of progress in molecular systems engineering, the design of self-assembling systems is poised to play a central role.
K. Eric Drexler, is an author, theoretical researcher, and policy advocate focused on emerging technologies and their consequences for the future. Noting that technological advances have caused some of the deepest transformations in human history, he studies emerging technologies with the power to cause future global transformations. Rather than concentrating solely on the immediate laboratory aspects of emerging technologies, where many scientists work in an array of narrow fields, Eric Drexler has chosen to focus on longer-term developments and their potential economic and social consequences, a broad area often neglected or overshadowed in the study of technological change. An advocate of long-term perspectives in policymaking, Eric writes and lectures widely on the implications of emerging technologies for our future. He is Founder, Chairman Emeritus, and Chairman of the Board of Advisors of Foresight Institute, a non-profit educational organization that works to help society prepare for emerging technological revolutions.
Personal technical site: e-drexler.com
|
Self-assembly at the University of Oregon
Darren W. Johnson, Assistant Professor, Inorganic, Organic, Supramolecular, and Materials Chemistry.
|
|
"Self-assembly refers to the ability of molecules to naturally join themselves together into larger structures due to the manners in which their geometric and binding structures complement one another. This feature, which is like a puzzle that puts itself together, is quite promising because it creates a final product that is more stable than the sum of its parts," Johnson explains.
From UO chemists create molecular 'claws' to trap arsenic atoms
NN: Please describe self-assembly for our readers. How does self-assembly play into your recent work on sequestering arsenic?
Self-assembly has been specifically defined as the spontaneous organization of molecules into well-defined aggregates. For example, the iron storage protein Ferritin is a self-assembled spherical protein shell composed of 24 identical protein subunits that stores a nanoparticle of iron oxides - basically rust wrapped in a protein capsule. Self-assembled aggregates such as these are typically held together by weak, reversible interactions. Since a large number of these weak interactions aid in forming the final self-assembled structure, it is usually more stable than the individual components that assemble it.
We are exploring whether the tool of self-assembly can be used to prepare compounds containing toxic metals, thereby housing the toxic metal in a very stable structure. We have designed a small organic molecule, three of which interact with two arsenic atoms to form a robust self-assembled arsenic-containing aggregate. Since the arsenic is used to assemble the final product, we hope to prove that this final product stores arsenic in a very stable form under a variety of conditions and in the presence of other competing metal ions. We are also attempting to develop design strategies to chelate a variety of other toxic metals.
NN: What are some of the potential applications for your "three-dimensional nanoscale architectures?"
We are applying our design strategy for forming self-assembled arsenic-containing compounds to the problem of specific metal chelation. By forming stable compounds that form only in the presence of the toxic metal ion, we hope that we not only are developing powerful chelators for that metal ion, but highly specific ones as well. This strategy could hold promise in the area of sensing and treating heavy metal poisoning in humans. We are also trying to apply what we are learning about the specific interactions between arsenic and small organic compounds to prepare improved materials for environmental arsenic remediation and sensing. We feel much of the knowledge we are gaining in forming self-assembled arsenic complexes can be translated to other toxic metal ions such as lead and mercury as well.
Another exciting feature of three-dimensional nanoscale assemblies is that they are often large enough to surround another molecule completely. Nature uses this principle of putting one molecule entirely within the confines of another molecule in many ways ranging from storing viral nucleic acids to the transport and storage of iron in mammals. The same principles can be used to store highly reactive species inside these "container molecules" so that they could be isolated and studied. There is also active research in using these nanoscale capsules as reaction vessels for applications in catalysis. A more distant application lies in the area of targeted drug-delivery. For instance, could a nanocage be developed that stores a drug molecule and delivers it to a specific target in the body?
Many Options for Molecular Manufacturing has been reprinted with permission from and courtesy of the Center for Responsible Nanotechnology.
Their Vision:
Advanced nanotechnology may build machines that are thousands of times more powerful—and hundreds of times cheaper—than today's devices. The humanitarian potential is enormous; so is the potential for misuse. The vision of CRN is a world in which molecular manufacturing is widely used for productive and beneficial purposes, and where malicious uses are limited by effective administration of the technology.
Their Mission:
CRN acts to raise awareness of the issues. We believe that even a technology as powerful as molecular manufacturing can be used wisely and well—but that without adequate information, unwise use will be far too common. The mission of CRN is to raise awareness of the issues presented by nanotechnology: the benefits and dangers, and the possibilities for responsible use.
Many Options for Molecular Manufacturing
Molecular manufacturing is the use of programmable chemistry to build exponential manufacturing systems and high-performance products. There are several ways this can be achieved, each with its own benefits and drawbacks. This essay analyzes the definition of molecular manufacturing and describes several ways to achieve the requirements.
Exponential Manufacturing Systems
An exponential manufacturing system is one that can, within broad limits, build additional equivalent manufacturing systems. To achieve that, the products of the system must be as intricate and precise as the original. Although there are ways to make components more precise after initial manufacture, such as milling, lapping, and other forms of machining, these are wasteful and add complications. So the approach of molecular manufacturing is to build components out of extremely precise building blocks—molecules and atoms, which have completely deterministic structures. Although thermal noise will cause temporary variations in shape, the average shape of two components with identical chemical structures will also be identical, and products can be made with no loss of precision relative to the factories.
The intricacy of a product is limited by its inputs. Self-assembled nanotechnology is limited by this: the intricacy of the product has to be built into the components ahead of time. There are some molecular components such as DNA that can hold quite a lot of information. But if those are not used—and even if they are—the manufacturing system will be much more flexible if it includes a programmable manipulation function to move or guide parts into the right place.
Programmable Chemistry: Mechanosynthesis
Chemistry is extremely flexible, and extremely common; every waft of smoke contains hundreds or thousands of carbon compounds. But a lot of chemistry happens randomly and produces intricate but uncontrolled mixtures of compounds. Other chemistry, including crystal growth, is self-templating and can be very precise, but produces only simple results. It takes special techniques to make structures using chemistry that are both intricate and well-planned.
There are several different ways, at least in theory, that atoms can be joined together in precise chemical structures. Individual reactive groups can be fastened to a growing part. Small molecules can be strung together like beads in a necklace. It's been proposed that small molecules can be placed like bricks, building 3D shapes with the building blocks fastened together at the edges or corners. Finally, weak parts can be built by self-assembly—subparts can be designed to match up and fall into the correct position. It may be possible to strengthen these parts chemically after they are assembled.
Mechanosynthesis is the term for building large parts by fastening a few atoms at a time, using simple reactions repeated many times in programmable positions. So far, this has been demonstrated for only a few chemical reactions, and no large parts have been built yet. But it may not take many reactions to complete a general-purpose toolbox that can be used in the proper sequence and position to build arbitrary shapes with fairly small feature sizes.
The advantage of a mechanosynthetic approach is that it allows direct fabrication of engineered shapes, and very high bond densities (for strength). There are two disadvantages. First, the range of molecular patterns that can be built may be small, at least initially—the shapes may be quite programmable, but lack the molecular subtlety of biochemistry. This may be alleviated as more reactions are developed. Second, mechanosynthesis will require rather intricate and precise machinery of a level that will be hard to build without mechanosynthesis. This creates a "bootstrapping" problem how to build the first fabrication machine. Scanning probe microscopes have the required precision, or one of the lower-performance machine-building alternatives described in this essay may be used to build the first mechanosynthesis machine.
Programmable Chemistry: Polymers and Possibilities
Biopolymers are long heterogeneous molecules borrowed from biology. They are formed from a menu of small molecules called monomers stuck end-to-end in a sequence that can be programmed. Different monomers have different parts sticking out the sides, and some of these parts are attracted to the side parts of other monomers. Because the monomer joining is flexible, these attractive parts can pull the whole polymer molecule into a "folded" configuration that is more or less stable. Thus the folded shape can be indirectly programmed by choosing the sequence of monomers. Nucleic acid shapes (DNA and RNA) are a lot easier to program than protein shapes.
Biopolymers have been studied extensively, and have a very flexible chemistry: it's possible to build lots of different features into one molecule. However, protein folding is complex (not just complicated, but inherently hard to predict), so it's only recently become possible to design a sequence that will produce a desired shape. Also, because there's only one chemical bond between the monomers, biopolymers can't be much stronger than plastic. And because the folded configurations hold their shapes by surface forces rather than strong bonds, the structures are not very stiff at all, which makes engineering more difficult. Biopolymers are constructed (at least to date) with bulk chemical processes, meaning that it's possible to build lots of copies of one intricate shape, but harder to build several different engineered versions. (Copying by bacteria, and construction of multiple random variations, don't bypass this limitation.) Also, reactants have to be flushed past the reaction site for each monomer addition, which takes significant time and leads to a substantial error rate.
A new kind of polymer has just been developed. It's based on amino acids, but the bonds between them are stiff rather than floppy. This means the folded shape can be directly engineered rather than emerging from a complex process. It also means the feature size should be smaller than in proteins, and the resulting shapes should be stiffer. This appears to be a good candidate for designing near-term molecular machine systems, since relatively long molecules can be built with standard solution chemistry. At the moment, it takes about an hour to attach each monomer to the chain, so a machine with many thousands of features would not be buildable.
There's a theorized approach that's halfway between mechanosynthesis and polymer synthesis. The idea is to use small homogeneous molecules that can be guided into place and then fastened together. Because this requires lower precision, and may use a variety of molecules and fastening techniques, this may be a useful bootstrapping approach. Ralph Merkle wrote a paper on it a few years ago.
A system that uses solution chemistry to build parts can probably benefit from mechanical control of that chemistry. Whether by deprotecting only selected sites to make them reactive, or mechanically protecting some sites while leaving others exposed, or moving catalysts and reactants into position to promote reactions at chosen sites, a fairly simple actuator system may be able to turn bulk chemistry into programmable chemistry.
Living organisms provide one possible way to use biopolymers. If a well-designed stretch of DNA is inserted into bacteria, then the bacteria will make the corresponding protein; this can either be the final product, or can work with other bacterial systems or transplanted proteins. (The bacteria also duplicate the DNA, which may be the final product.) However, this is only semi-controlled due to complex interactions within the bacterial system. Living organisms dedicate a lot of structure and energy to dealing with issues that engineered systems won't have to deal with, such as metabolism, maintaining an immune system, food-seeking, reproduction, and adapting to environmental perturbations. The use of bacteria as protein factories has already been accomplished, but the use of bacteria-produced biopolymers for engineered-shape products has only been done in a very small number of cases (e.g. Shih's recent octahedra [PDF]; in this case it was DNA, not protein), and only for relatively simple shapes.
Manufacturing Systems, Again
Now that we have some idea of the range of chemical manipulations, we can look at how those chemical shapes can be joined into machines. Machines are important because some kind of machine will be necessary to translate programmed information into mechanical operations. Also, the more functions that can be implemented by nano-fabricated machines, the fewer will have to be implemented by expensive, conventionally manufactured hardware.
A system with the ability to build intricate parts by mechanosynthesis or small building blocks probably will be able to use the same equipment to move those shapes around to assemble machines, since the latter function is probably simpler and doesn't require much greater range of motion. A system based on biopolymers could in theory rely on self-assembly to bring the molecules together. However, this process may be slow and error-prone if the molecules are large and many different ones have to come together to make the product. A bit of mechanical assistance, grabbing molecules from solution and putting them in their proper places while protecting other places from incorrect molecules dropping in, would introduce another level of programmability.
Any of these operations will need actuators. For simple systems, binary actuators working ratchets should be sufficient. Several kinds of electrochemical actuators have been developed in recent months. Some of these may be adaptable for electrical control. For initial bootstrapping, actuators controlled by flushing through special chemicals (e.g. DNA strands) may work, although quite slowly. Magnetic and electromagnetic fields can be used for quite precise steering, though these have to be produced by larger external equipment and so are probably only useful for initial bootstrapping. Mechanical control by varying pressure has also been proposed for intermediate systems.
In order to scale up to handle large volumes of material and make large products, computational elements and eventually whole computers will have to be built. The nice thing about computers is that they can be built using anything that makes a decent switch. Molecular electronics, buckytube transistors, and interlocking mechanical systems are all candidates for computer logic.
High Performance Products
The point of molecular manufacturing is to make valuable products. Several things can make a product valuable. If it's a computer circuit, then smaller component size leads to faster and more efficient operation and high circuit density. Any kind of molecular manufacturing should produce very small feature sizes; thus, almost any flavor of molecular manufacturing can be expected to make valuable computers. A molecular manufacturing system that can make all the expensive components of its own machinery should also drive down manufacturing cost, increasing profit margins for manufacturers and/or allowing customers to budget for more powerful computers.
Strong materials and compact motors can be useful in applications where weight is important, such as aerospace hardware. If a kilowatt or even just a hundred watt motor can fit into a cubic millimeter, this will be worth quite a lot of money for its weight savings in airplanes and space ships. Even if raw materials cost $10,000 a kilogram, as some biopolymer ingredients do, a cubic millimeter weighs about a milligram and would cost about a penny. Of course this calculation is specious since the mounting hardware for such a motor would surely weigh more than the motor itself. Also, it's not clear whether biopolymer or building-block styles of molecular manufacturing can produce motors with anywhere near this power density; and although the scaling laws are pretty straightforward, nothing like this has been built or even simulated in detail in carbon lattice.
Once a process is developed that can make strong programmable shapes out of simple cheap chemicals, then product costs may drop precipitously. Mechanosynthesis is expected to achieve this, as shown by the preliminary work on closed-cycle mechanosynthesis starting with acetylene. No reaction cycle of comparable cost has been proposed for solution chemistry, but it seems likely that one can be found, given that some polymerizable molecules such as sugar are quite cheap.
Future Directions
This essay has surveyed numerous options for molecular manufacturing. Molecular manufacturing requires the ability to inject programmability for engineering, but this can be done at any of several stages. For scalability, it also requires the ability to build nanoscale machines capable of building their duplicates. There are several options for machines of various compositions and in various environments.
At the present time, no self-duplicating chemical-building molecular machine has been designed in detail. However, given the range of options, it seems likely that a single research group could tackle this problem and build at least a partial proof of concept device—perhaps one that can do only limited chemistry, or a limited range of shapes, but is demonstrably programmable.
Subsequent milestones would include:
- Not relying on flushing sequences of chemicals past the machine
- Machines capable of general-purpose manufacturing
- Structures that allow several machines to cooperate in building large products
- Building and incorporating control circuits
Once these are achieved, general-purpose molecular manufacturing will not be far away. And that will allow the pursuit of more ambitious goals, such as machines that can work in gas (instead of solution) or vacuum for greater mechanical efficiency. Working in inert gas or vacuum also provides a possible pathway (one of several) to what may be the ultimate performer: products built by mechanosynthesis out of carbon lattice.
Read comments, debate, and updates.
What Is Happening to the Funding for Commercializing Nanotechnology?
|
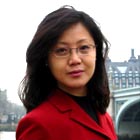 |
The industries that nanotechnology will likely have a disruptive effect on in the near term include the following:
(Amounts are Billions of US Dollars)
$1,700 |
Healthcare |
$600 |
Long Term Care |
$550 |
Electronics |
$550 |
Telecom |
$480 |
Packaging |
$450 |
U.S. Chemical |
$460 |
Plastics |
$182 |
Apparel |
$180 |
Pharmaceutical |
$165 |
Tobacco |
$100 |
Semiconductor |
$92 |
Hospitality / Restaurant |
$90 |
US Insurance |
$83 |
Printing |
$80 |
Corrosion Removal |
$57 |
US Steel |
$43 |
Newspaper |
$42 |
Diet Supplement |
$40 |
Diet |
$32 |
Publishing |
$30 |
Catalysts |
$27 |
Glass |
$24 |
Advertising |
$18 |
Cosmetics |
$13 |
Chocolate |
$10 |
Battery |
$5 |
Blue Jeans |
$4 |
Khakis |
$2.8 |
Fluorescent Tagging |
|
Our Review
The Superswarm Interview
The Superswarm Option
Nanoveau - This column will cover the science, the speculation, and (occasionally) the politics of nanotechnology and related topics. If you want to know what nanotech is about, and how and why it will change everything we know-Nanoveau is for you.
|
|
Within the past few weeks I have heard two pieces of very disturbing news from the investment industry that could negatively impact commercialization of nanotechnology in the United States.
The first piece of disturbing news is that there is an initiative by the Bush Administration to cut funding to the National Institute of Standards & Technology (NIST) (formerly known as the National Bureau of Standards (NBS)). Since I have done some research work at and with NIST, I admit I do have a personal interest in this excellent lab's welfare. NIST, since it is overseen by the Department of Commerce, is never quite perceived as the technical powerhouse of a government lab that it is, mainly due to misconceptions of what type of research it does. There are excellent scientists there who do research at this government lab that in many cases have very little to do with Standards and more to do with Technology and much of it is nanotechnology. Many do not perceive Commerce as having to do with technology and that may be because NIST does not have the word "laboratory" in its name like Sandia, Oak Ridge, Argonne, Fermi, Jet Propulsion, or Brookhaven. Hence it suffers from not being recognized as a heavyweight contender in government labs. Forgive me if I have left out a few government labs in this list because there are certainly others but I am just making a point here. Many do not even realize that NIST has a neutron reactor on site that is visited by scientists all over the world who collaborate with the NIST scientists. Of course, scientists all over the world also collaborate with the non-reactor scientists as well. I beg the environmentalist please do not go up in arms against NIST now because there is a reactor on the grounds. NIST has never hidden this fact from the public and it goes out of its way to meet government safety standards in shielding and safe operating protocols.
However, my bigger concern with this first piece of disturbing news is that the funding is also going to be cut from the Advanced Technology Program (ATP), another underrated and under-marketed program in the federal government, overseen by NIST and headquartered on its grounds in Gaithersburg, Maryland. Few know that the ATP is one of the only federal government programs that exist to fund commercialization of early stage technology. Most federal funding programs, such as the 21st Century Nanotechnology Act, fund early stage research and development at the university and government lab levels at pre-commercialization stage. However, ATP is a program that bridges that funding gap between seed and early stage and VC funding to commercialize the technology. Most people do not even know about ATP besides that it also stands for an important molecule (adenosine triphosphate) in the energy conversion cycle in the human body.
NIST is one of the government agencies on the list for federal nanotechnology funding, and the ATP is under NIST's umbrella.
So, the Bush Administration signs on December 3rd, 2003 into law The 21st Century Nanotechnology Act to fund research and development of nanotechnology on the university and government lab levels but then begins initiatives to cut the legs off of the very programs that can help commercialize these technologies? Does this make sense? I understand that we need to allocate funding to military and Homeland Security initiatives. But what about the nanotechnologies that have military and Homeland Security applications, such as sensors for biological and chemical agents and weapons, one of our biggest security fears? How are we going to commercialize these technologies without funding?
Wait… It gets worse.
The second piece of disturbing news is that the Small Business Investment Company (SBIC) program under the Small Business Adminstration (SBA) is undergoing major changes. Typically the SBIC program offers two options for funding: Participating Securities and Debentures. The Participating Securities, as opposed to the more traditional Debentures or debt based funding, has been stalled. The Debentures option is still active. Funding by debentures require companies to make semi-annual interest payments, much like balloon type mortgages. No new licenses for Participating Securities SBIC's will be issued as of end of September 30, 2004. For the past 12 months, steps have been taken to curtail the Participating Securities part of the SBIC program. Strangely enough, this started around the same time the 21st Century Nanotechnology Act was signed into law.
The SBIC program was created by the Small Business Investment Act of 1958 to bridge the gap between entrepreneurs' need for capital and traditional financing sources. This act was designed to stimulate and supplement the flow of private equity capital and long-term funds which small businesses need for financing their operations and for growth, expansion, and modernization.
Since 1979, roughly 2/3 of the financing for VC funds comes from institutional investors, such as pension funds, insurance companies and university endowments. This was because in 1979, the Labor Department liberalized the interpretation of the "prudent man" rule under ERISA (the Employee Retirement Income Security Act) which changed the composition of investors in the venture capital industry and increased the total flow of funds into the industry. This act sparked the venture capital industry in the U.S. which has made us the haven for small businesses, giving them a chance to succeed like in no other country and making it possible for many to achieve their dreams.
In 1991 the SBA appointed an Advisory Commission to review SBIC industry performance. The Advisory Commission determined that SBIC's had a significant role to play in building the U.S. economy. The principle features of their outlined program changes were embodied in legislation called the "Small Business Equity Enhancement Act of 1992." This created a new form of SBA leverage known as "Participating Securities." This act increased the mount of leverage available to an SBIC to $90 million, and introduced the idea of the SBA taking an equity stake in a SBIC investment firm as opposed to offering more traditional Debentures or debt as leverage.
According to the SBA, from 1994-2002, SBIC's provided 8% of all venture financing dollars (which comes to $23.7 billion out of $280.5 billion total), 64% of all seed financing dollars, and 62% of all venture financings by actual number. In 2002, over 27% of those funds were allocated to low and moderate income areas. Examples of well known (and successful) companies that SBIC's have helped fund are Amgen, Apple Computer, FedEx, DoubleClick, Staples, Jenny Craig, Intel, Sun Microsystems.
Since then, the SBIC program has been looked upon by the rest of the world with envy because the U.S. government has enough money to invest in its own businesses, which has helped to stimulate its economy. So much so that the UK Government has implemented its own SBIC program as of end of 2003, called the Equity Capital Fund (ECF), which is modeled after the U.S. SBIC program.
The Participating Securities program is ideal for early stage companies with no significant revenues, as would be the case for nanotechnology startups. Debentures are not appropriate for early stage technology investing because these types of companies do not produce revenues enough to enable them to make the semi-annual interest payments. The Participating Securities program is rumored to be overhauled by late 2006 or early 2007 to take a much larger equity stake in return for funds. In the meantime, there is no program appropriate for investing in early stage nanotechnology startups.
It is true that the SBIC program has lost much money in the past decade due to bad investments and the economy, causing many approved SBIC's to fail …what VC or Investment Bank hasn't? However, why does the current Participating Securities program have to be curtailed until its overhaul without a stop-gap solution in the interim? Do we really know how long it will take to overhaul the current Participating Securities program? What are aspiring nanotech SBIC's to do in the meantime? Why does it seem that funding commercialization of nanotechnology versus research and development of nanotechnology is not as important? If the federal government believes that commercialization of nanotechnology is not yet important and that nanotechnology is still in its very nascent stages, it just has to look around for confirmation that that is not true.
In addition, if the U.S. VC firms are not given any more access to cheap government money, they will not invest in commercializing nanotechnology, and someone else will. Did you know Japanese banking firms like Sumitomo are already in our backyard on the West Coast looking for nanotechnology to invest in?
We will have much good research funded by the $3.7 billion 21st Century Nanotechnology Act, but other countries, not the U.S., will fund the commercialization of that research and make the money from it. In effect, the U.S. will become the nanotechnology incubator for the rest of the world. If we slow down our investment in nanotechnology, the U.S. will surely lose its slim lead in technology in the world, and its status as the richest country in the world. In the long run, the U.S. may lose economic and political influence while other countries copy our successful investment models to beat us at our own game.
If you have a passion for nanotechnology, I strongly suggest you contact your nearest Congressman and find out if there is anything you and they can do to provide a stop-gap solution for NIST and ATP funding, for obtaining SBIC licenses, and somehow continue to provide a form Participating Securities funding, even if in a somewhat limited form, while they overhaul the current program instead of stopping it altogether while they figure it all out. It would be a terrible thing to watch nanotechnology research flourish in the U.S. (with taxpayer money) but then have to watch some other country make the money from it because we, as a country, cannot afford right now to invest in commercialization. I think it is time to bring in another Advisory Commission, and this overhaul needs to be done quickly.
Stay tuned for next month's article.
Dr. Pearl Chin has an MBA from Cornell, a Ph.D. in Materials Science and Engineering from University of Delaware's Center for Composite Materials and B.E. in Chemical Engineering from The Cooper Union.
Dr. Chin specializes in advising on nanotechnology investment opportunities. She is also CEO of Red Seraphim Consulting where she advises investment firms and startup firms on the business strategy of nanotechnology investments. She was Managing Director of the US offices and co-Managing Director of the London offices of Cientifica. Prior to that, she was a Management Consultant with Pittiglio Rabin Todd & McGrath (PRTM)'s Chemicals, Engineered Materials and Packaged Goods group.
Dr. Chin will be advising the Cornell University JGSM's student run VC fund, Big Red Venture Fund (BRVF), on investing in nanotechnology.
She is a Senior Associate of The Foresight Institute in the US and was the US Representative of the Institute of Nanotechnology in the UK. She was an alternate finalist for a Congressional Fellowship with the Materials Research Society.
She was also a Guest Scientist collaborating with the National Institute of Standards & Technology (NIST) Polymer Division's Electronic Materials Group under the US Department of Commerce.
Dr. Chin is a US Citizen, born and raised in New York City.
She can be contacted here.
|
Quotes
Simply put, we're talking about collections of objects that put themselves together. Imagine holding a box containing a jigsaw puzzle, giving the box a shake, and peeking inside to find that the puzzle had assembled itself! While such behavior would be shocking in a jigsaw puzzle, a little reflection reveals that it's not so surprising to find self assembling systems in the natural world. After all, no one put you together did they? Biological systems as well as a variety of inorganic physical systems exhibit self assembling or self ordering behavior. Drawing on these systems for inspiration, scientists from numerous disciplines, chemistry, physics, biology, engineering, and mathematics, to name a few, have begun to investigate the self assembly process in hopes of learning to design and control the behavior of self assembling systems. Much of this work is motivated by recent advances in micro- and nanoscale science. On one hand, fabrication methods in micro- and nanoscience allow for batch processing. That is, we have the ability to make many copies of the same device simultaneously. How do we design these devices so that they spontaneously assemble themselves into a useful working structure? On the other hand, traditional fabrication methods are limited in resolution. To make smaller structures, i.e., true nanoscale structures, requires the development of new methods. Taking their cue from nature, coaxing nanostructures into self assembling is an avenue many scientists are exploring. Ultimately, a deeper understanding of self assembly may shed light on the nature of life itself.
What is self assembly?
"Molecular self-assembly is a strategy for nanofabrication that involves designing molecules and supramolecular entities so that shape-complementarity causes them to aggregate into desired structures. Self-assembly has a number of advantages as a strategy: First, it carries out many of the most difficult steps in nanofabrication--those involving atomic-level modification of structure--using the very highly developed techniques of synthetic chemistry. Second, it draws from the enormous wealth of examples in biology for inspiration: self-assembly is one of the most important strategies used in biology for the development of complex, functional structures. Third, it can incorporate biological structures directly as components in the final systems. Fourth, because it requires that the target structures be the thermdynamically most stable ones open to the system, it tends to produce structures that are relatively defect-free and self-healing."
George M. Whitesides, Department of Chemistry, Harvard University, Self Assembly and Nanotechnology
Physicists in Ireland have developed a novel technique that allows "hands-free" manipulation of mesoscale device components for the first time. The method employs electric fields to direct the assembly of the components into integrated systems and could be an alternative to conventional techniques for making electronic devices.
Self-assembly made easy
Researchers at the Technion-Israel Institute of Technology have used the self-assembly of DNA molecules to build electronic devices from carbon nanotubes. The DNA acts as a scaffold for positioning a single-walled carbon nanotube at the heart of a field-effect transistor, as well as a template for the metallic wires connecting the device.
DNA self-assembles nanotube transistor
"Much like proteins which must fold into complex structures in order to function properly, we have designed new materials that also form complex structures through the process of self-assembly."
Chad A. Mirkin, director of Northwestern's Institute for Nanotechnology
From Our Molecular Future: How Nanotechnology, Robotics, Genetics, and Artificial Intelligence Will Transform Our World, by Douglas Mulhall:
-
What happens to the monetary system when everyone is able to satisfy his own basic material needs at very low cost?
-
How would we use cash when digital manufacturing makes it impossible to differentiate a counterfeit bill or coin from the real thing?
-
What happens to fiscal policy when digital information, moving at light speed, is the major commodity?
-
How fast will monetary cycles move compared to, say, the ten- or twenty-year cycles of the late twentieth century, when products and patents go out of date in a matter of months instead of years?
-
What happens when we don't have to worry about trade or social services for our basic needs, because most of what we need is provided locally with digital manufacturing, and the biggest trade is in information?
-
How do we control the excesses of the ultrarich, the overabundance of the molecular assembler economy, and the challenge to intellectual property laws created by intelligent, inventive machines?
-
What happens if half of all jobs are made redundant every decade?
-
What happens to the War on Drugs when there's no import, export, or transport of contraband because drugs can be manufactured in a desktop machine using pirated software downloaded from the Internet?
-
What happens to democratic controls when individuals can get as rich as small governments in a year or so?
-
What's the relevance of insurance if many things are replaceable at very low capital cost, but liabilities from software are potentially unlimited?
-
How should organized labor react when molecular assemblers and intelligent robots eliminate most manufacturing jobs?
-
What is the nature of work going to be?
-
What happens to land prices when an individual can build a tropical farm under a bubble in North Dakota, and get there from New York in an hour?
-
What happens when everyone can go everywhere, whenever they want, and work from wherever they want?
Return to Top
Useful Links
Nano Self-Assembly: An NSTI Executive Briefing
DNA 'Velcro' binds nanoparticles
Molecular Self-Assembly Research Leads to Alzheimer's Discovery
DNA Computing by Self-Assembly
Exploring Self-Assembly Processes Using Magnetic PDMS Tiles
DNA self assembly by Ned Seeman
Self-assembly of Microspheres
Centre for Self-Organising Molecular Systems
Self-Assembled Monolayers for Diagnostics & Sensing
Return to Top
IN THE NEXT ISSUE
Issue #19 will cover Patents. It will land in your mailbox January 3rd, 2005.
Infamous Quotes:
"This 'telephone' has too many shortcomings to be seriously considered as a means of communication. The device is inherently of no value to us." Western Union internal memo, 1876
"Heavier-than-air flying machines are impossible." - Physicist and mathematician Lord Kelvin, President of the British Royal Society, 1895
"Everything that can be invented has been invented." - Charles H. Duell, Director of U.S. Patent Office, 1899
"There is no likelihood man can ever tap the power of the atom." - Robert Milikan, Nobel Laureate in Physics, 1923
"Theoretically, television may be feasible, but I consider it an impossibility-a development which we should waste little time dreaming about." - Lee de Forest, inventor of the cathode ray tube, 1926
"I think there is a world market for maybe five computers." IBM's Thomas Watson, 1943
"Landing and moving around on the moon offer so many serious problems for human beings that it may take science another 200 years to lick them." - Science Digest, August 1948
"Computers in the future may weigh no more than 1.5 tons." Popular Mechanics, 1949
"There is no reason anyone would want a computer in their home." Ken Olsen, Digital Equipment Corp, 1977
And the lesson is? It's a tough game to call.
Need advice? Check out NanoStrategies
© Copyright 1999-2004 7thWave, Inc. All rights reserved. More information on copyright, and disclaimers regarding the content of this newsletter can be found here
|